Science
Related: About this forumGreat Science in a Dark Dictatorship: Separation of Americium from Lanthanides.
The paper I'll discuss in this post is this one: Highly Efficient Removal of Americium(III) from High-Level Waste Using Novel Phenanthroline Diamide Ligands Mariia V. Evsiunina, Peter I. Matveev, Paulina Kalle, Pavel S. Lemport, Nane A. Avagyan, Konstantin A. Lyssenko, Alexandr V. Belousov, Vladimir G. Petrov, Yuri A. Ustynyuk, Igor P. Gloriozov, and Valentine G. Nenajdenko, Industrial & Engineering Chemistry Research 2024 63 (44), 19234-19248.
One wonders if science can survive in an atmosphere of vile dictatorships, which is of course, now relevant to the United States under His Maggotcy King Elon and his idiot sock puppet. Thus I take some solace in this excellent paper in service to the future of humanity, should humanity have a future, science that took place under King Vladimir I of Russia, a worthy successor to Ivan the Terrible.
I see Americium, considered as one of the so called "minor actinides," as a potentially valuable nuclear fuel. It is said that each ton of used nuclear fuel, upon fresh removal from a nuclear reactor, contains about 100 grams per ton of americium. These quantities increase with time of storage because of the decay of 241Pu, an isotope of plutonium that has a half life of 14.290 years and decays by beta emission to 241Am. The United States has accumulated about 85,000 tons of valuable used nuclear fuel, as it remains the largest producer of clean nuclear energy in the world (soon to be superseded by China). Much of it has been stored for decades, so it is likely that the quantities of americium in US used nuclear fuels is greater than (0.100 kg * 85000) = 8500 kg = 8.5 tons.
This is probably facile for bulk separations, owing to the fact, that americium, like the lanthanides, does not form volatile fluorides, whereas the lighter actinides, uranium (which makes up the bulk of used fuel, generally on the order of 95%), neptunium, and plutonium all form volatile fluorides, as do many fission products, such as technetium, ruthenium, rhodium, molybdenum, zirconium, to name a few others. Thus in the volatile fluoride method of reprocessing, the concentrated residue will mostly contain lanthanides, americium, curium, and elements like barium and strontium.
Some time ago I gave the critical masses of americium isotopes in this space, which is here: Critical Masses of the Three Accessible Americium Isotopes.
One of the interesting things about Americium as a potential fuel is its high neutron multiplicity in a fast neutron spectrum, which makes it possible to recover all of the neutrons that went into making it ( greater than 3, in the case of 241Am) allowing for excess neutrons for transmutation of other actinides or breeding in the case of 238U, for making more plutonium to save the world.
In general, the idea of separating americium does not, regrettably, is only subject to removing it to reduce the heat load of used nuclear materials, but in a wiser world than the one in which we live, it would be utilized as a fuel. It is likely that we can easily obtain well over 100 critical masses of americium, particularly if alloyed with other actinides. In a breed and burn setting, as a "starter" fuel, it might power reactors that can run for decades without refueling.
Thus my interest in this paper. From the introduction:
Nuclear power is an essential source of energy with minimal carbon dioxide emissions, which is of great importance in connection with climate change issues. (1,2) Expanding the life cycle of the main material, enriched uranium dioxide, the main part of nuclear fuel, is a key issue for further sustainable development of nuclear power. The final stages of the appeal require solving the main problem, the formation of large volumes of spent nuclear fuel (SNF). The PUREX process is currently used in industrial scale for the isolation of uranium and plutonium from SNF. (3,4) Nevertheless, the remaining HLW still contains minor actinide elements, such as Am, Cm, and Np, which represent a significant environmental concern due to their high radiotoxicity. (5) Therefore, further processing of HLW is necessary to be consistent with the concept partitioning and transmutation which consists of separating radionuclides into groups (partitioning) and further converting them into radionuclides with shorter half-lives or stable nuclides (transmutation) using fast neutron reactors. (6−8)
Americium is represented by two isotopes, Am-243 (t1/2 = 7400 years) and Am-241 (t1/2 = 430 years), which makes it one of the main sources of radiotoxicity in the long run. Therefore, americium is one of the most hazardous components of HLW. Moreover, its inclusion in waste composition for deep geological disposal will result in a significant increase in the volume of radioactive waste for vitrification. Also, the presence of americium in HLW leads to increased heat generation, which is potentially very dangerous. The transmutation of americium isotopes will solve these problems. However, for this aim, it is essential to separate Am(III), Cm(III), and lanthanides(III) (Ln(III)). Lanthanides have large neutron cross sections and are present in HLW in higher concentrations than americium and curium. Curium-242 and curium-244 have high neutron emission, which sharply reduces the transmutation efficiency. (9,10) That is why it is important to separate Am(III), Cm(III), and Ln(III), which is quite tricky due to the similarity of their chemical properties and close ionic radii...
Some figures from the text:
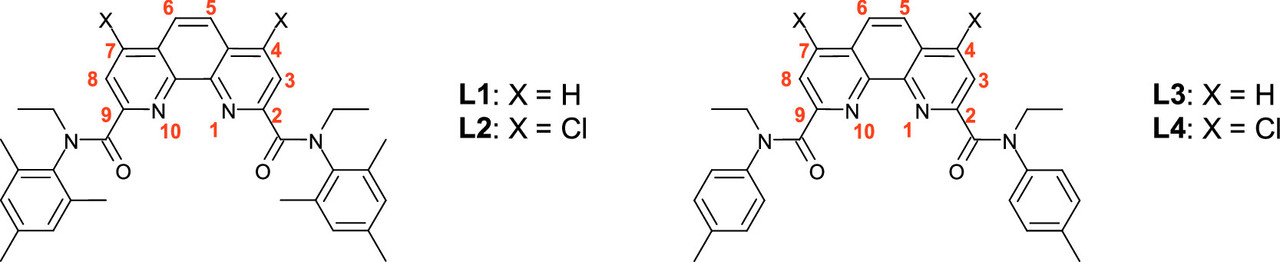
The caption:
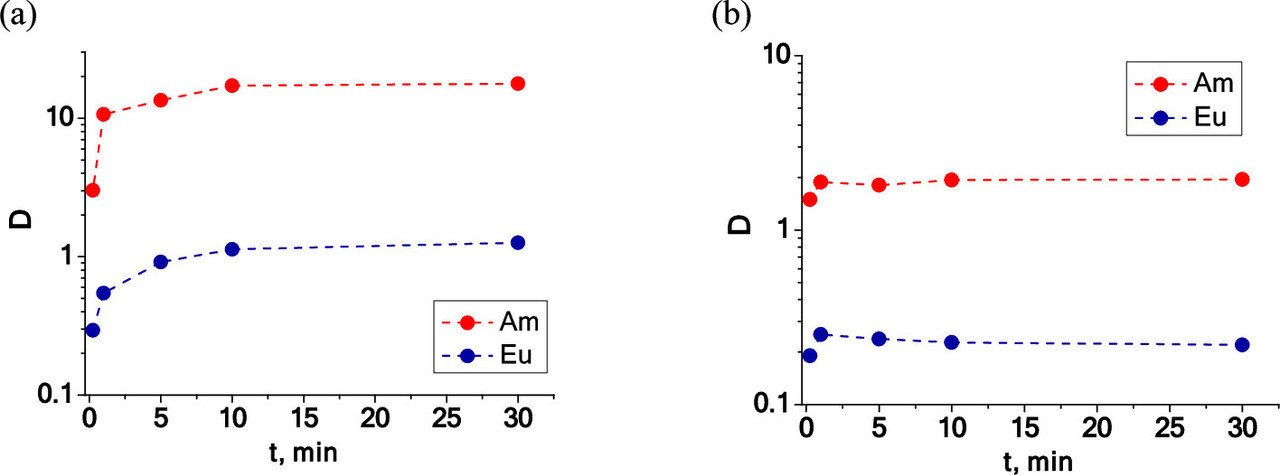
The caption:
"F-3," the solvent, is "3-nitrobenzotrifluoride" (a trade name for trifluoromethyl-3-nitrobenzene). It is notable that the separations from europium are particularly important because europium, among the lighter lanthanides has a particularly high neutron capture cross section, although in long burnups (as I envision for "breed and burn" systems), this tends to deplete this element by transmuting it into gadolinium.
The distribution factors for all of the lanthanides is given in the following table. In general, in fission in the used nuclear fuels now available for use by humanity, in thermal light water reactors, which dominate the world inventory, do not generate the transgadolinium lanthanides, although one might imagine, for very large burnups, again in a "breed and burn" setting, one might see, via neutron capture reactions, small amounts of holmium and the important and valuable element dysprosium formed, the latter being an element that is very important as a low concentration additive in the long terms stability of neodymium-iron-boride magnets used in generators.
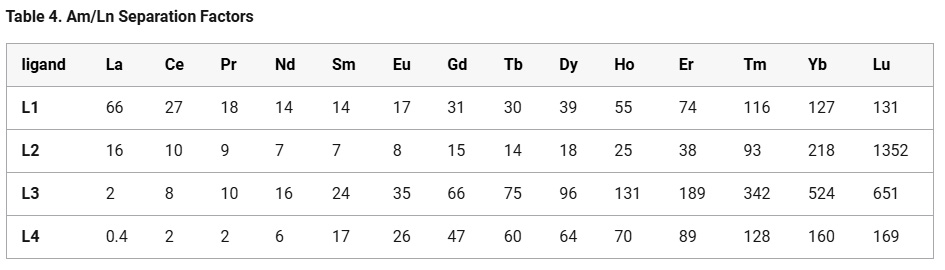
The effect of acid concentration in the aqueous phase on the distribution between europium and americium:
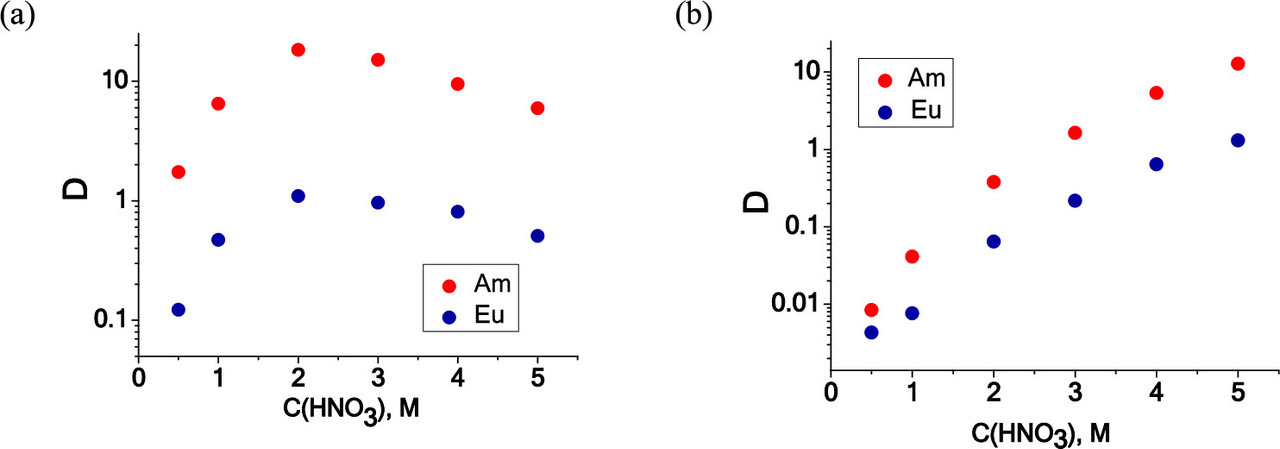
The caption:
It should be noted that with appropriate reducing agents, europium and americium can be separated by exploiting the lower oxidation state available for europium (but not, generally, the other lanthanides) in aqueous solution, the +2 oxidation state, where europium behaves very much like a congener of strontium and barium, and thus forms insoluble sulfates (and, in neutral or slightly basic solutions, insoluble carbonates.)
A graphic on the separation factors for all the lanthanides from americium for examples of the extraction agents. Note that these graphics are logarithmic on the ordinate:
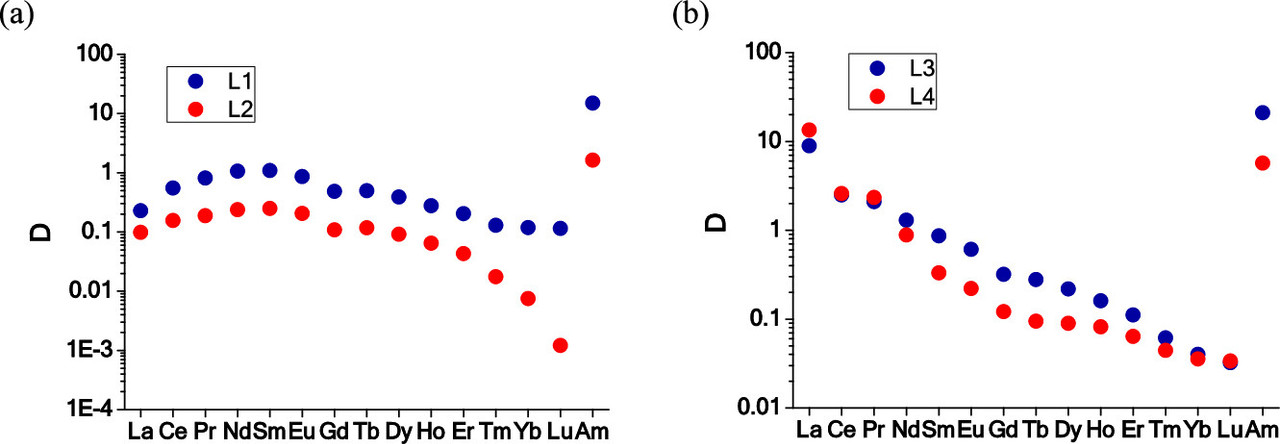
The caption:
The following graphic shows, the distribution factors of a range of lanthanides and some other fission products. (Zirconium forms a volatile fluoride; palladium does not. However in used nuclear fuels, palladium is present in metallic inclusions of the "noble metals," ruthenium, rhodium, and palladium with some technetium and molybdenum found in these inclusions. These inclusions tend not to dissolve in nitric acid.) Again, note that the ordinate is logarithmic:
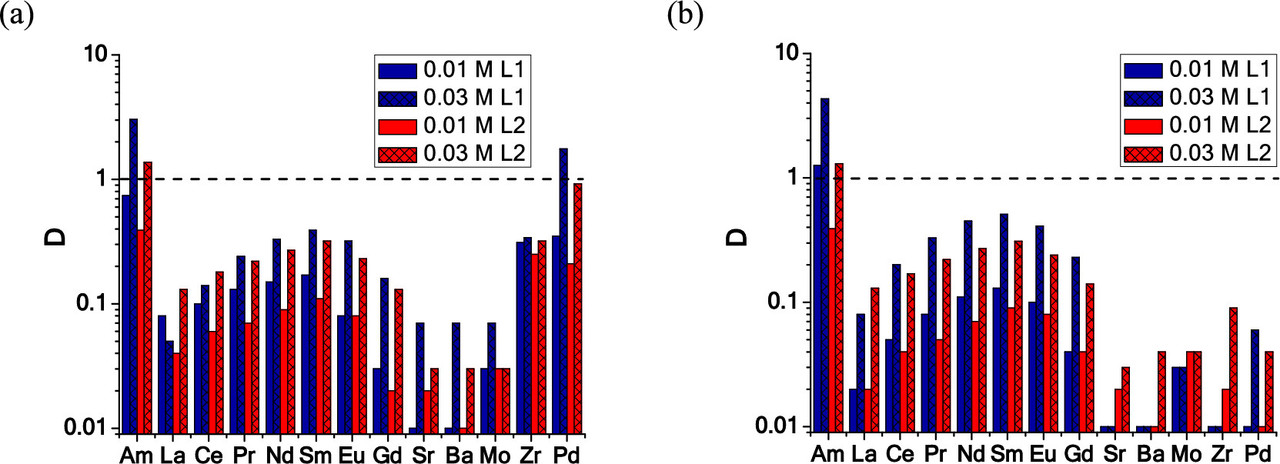
The caption:
Some data on the radiation stability of the ligand:
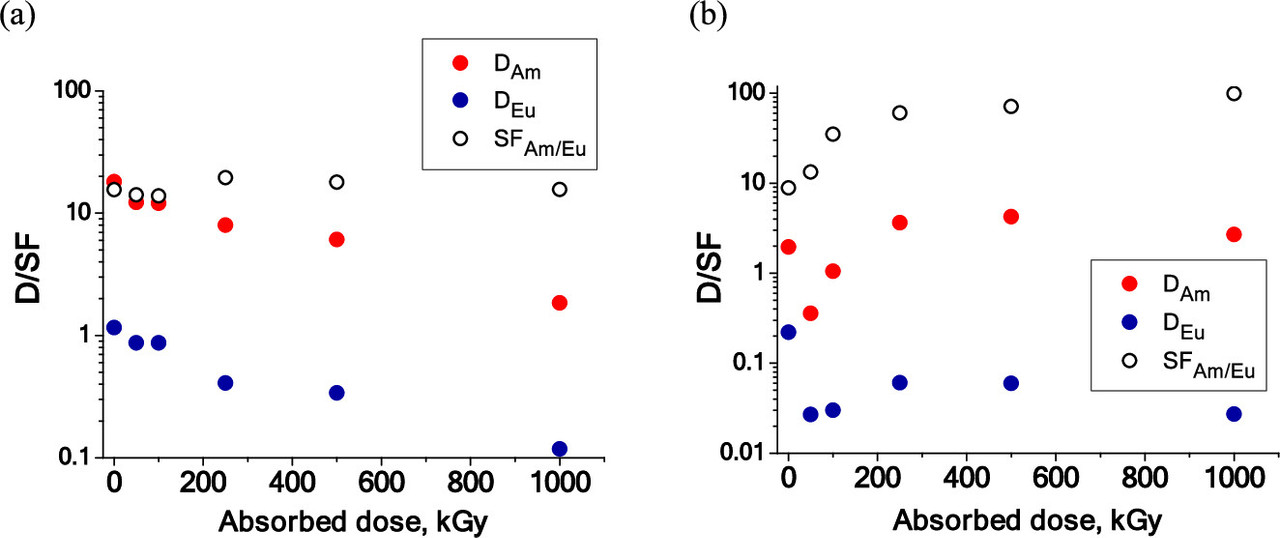
The caption:
From the paper's conclusion:
A detailed study of the extraction and coordination properties of two alkyl-aryl phenanthroline diamide ligands differing in the presence of substituents at the 4,7-position of the phenanthroline core was performed. The introduction of methyl groups into the ortho-positions of the aryl fragment at the amide nitrogen atoms leads to a drastic change in the extraction of lanthanides(III). As a result, record values for the separation factors of Am(III) and light lanthanides(III) at 3 mol/L HNO3 were observed: SFAm/La = 66 for L1 and 16 for L2 and SFAm/Ce = 27 for L1 and 10 for L2. The effectiveness of these extraction systems in separating Am(III) from the HLW imitator solution containing 10.7 g/L lanthanide(III), 0.5 g/L Pd, and 0.2 g/L Zr was demonstrated. This is the first example of alkyl-aryl DAPhen ligands that allow for the separation of Am(III) from light lanthanides(III) under strongly acidic conditions...
I trust you have enjoyed one of the last weekends under a sane government. Watch out for the future.

Easterncedar
(3,736 posts)And enjoy pretending I can understand them. The occasional glimmer feels pretty good.
NNadir
(34,964 posts)...think I can, understand.
It's generally a worth exercise, as eventually, after a time, things do sink in.
Thanks again.
ProfessorGAC
(71,160 posts)One of my first projects in private industry was developing a process to make a polymeric diameter (amidoamine, too).
The intended use was >>>>>>>>> ore flotation & floculation!
I hadn't thought about it for a long, long time until I read your post!
NNadir
(34,964 posts)...probably here - although I don't remember - and on other websites to which I was invited. The longest was on Barry Brook's website, "Is Uranium Exhaustible?" in which I discussed the geological uranium cycle through the mantle, to the crust, and ultimately the oceans, from which it can be recovered, albeit at a price higher than domestic ores. I'm not sure it's still available; I wrote in 2015. I note that the same technology can be used to remove uranium from groundwater that percolates through uranium ores (NORM - Naturally Occurring Radioactive Materials). Theoretically the uranium could be recovered for use.
I have a rather long list of papers on the topic in my electronic library.
I also wrote a piece on Rod Adams' website Atomic Insights: On Plutonium, Nuclear War, and Nuclear Peace
I believe it would be impossible for humanity to consume all of the uranium cycling through the crust, through rivers and ultimately into the oceans, with the possible caveat that the rate of consumption would matter. It does not seem unreasonable to assume that enough uranium leaches out of the crust to continuously supply the world's energy demand at say, 1000 EJ/year indefinitely, albeit with the requirement that 238U is converted in the fast neutron spectrum to 239Pu and beyond.
I believe you may be the first person with whom I have communicated to have actually worked on these materials.
Thanks for your comment.